The Impact of Bariatric Surgery on Calcium and Vitamin D Status
This activity expired June 1, 2022.
Tracy Martinez, RN, BSN, CBN
Tracy Martinez, RN, BSN, CBN, is Program Director of Wittgrove Bariatric Center in La Jolla, California
A Message from the Department Editor
Dear Colleagues:
It is with great pleasure that I introduce the third article in a four-part series highlighting key areas affected by inadequate nutrition status in the postbariatric surgery patient. In this article, we focus on the identification, treatment, and prevention of calcium and vitamin D deficiencies in both pre- and postoperative bariatric surgical patients. After decades of performing bariatric and metabolic surgery, we continue to see vitamin deficiencies in our patients following bariatric surgery. The vast majority of these deficiencies can and should be avoided, most importantly for patient health and well being, but also to protect our specialty’s reputation in the medical field as a whole. Proper vitamin supplementation patient education, surveillance, and protocols are the entire team’s responsibility to support optimal patient outcomes.
Cassie Story, RDN, has done an outstanding job outlining the risks and clinical findings of inadequate supplementation of calcium and vitamin D, bariatric surgery patients, as well as appropriate supplementation and patient assessment.
I hope you enjoy this article and take advantage of the continuing education credit. Please send me your comments and ideas for future articles so we can build a collaborative platform for ongoing education.
My best to you,
Tracy Martinez, RN, BSN, CBN
by Cassie I. Story, RDN
Ms. Story has a private practice in Scottsdale, Arizona.
Funding: This continuing education activity was supported by Bariatric Advantage (Aliso Viejo, California).
Disclosures: Cassie I. Story, RDN, is the Director of Nutrition Education for Bariatric Advantage.
Abstract: There is an urgent need for science-based nutrition recommendations following bariatric surgery. While evidence indicates that bariatric surgery is the most effective treatment option for someone affected by obesity, the increase in reported nutrition deficiencies and complications due to inadequate nutrition status postoperatively is cause for alarm. In Part 3 of a four-part Core 4 educational series on identifying, preventing, and treating nutrition deficiencies in preoperative and postoperative bariatric surgery patients, we focus on calcium and vitamin D and their implications in bone health. In this continuing education article, we review how bariatric surgery can impact calcium absorption and affect vitamin D status, discuss pre- and postoperative screening for calcium and vitamin D deficiency, and provide nutrition treatment recommendations for patients who have had bariatric surgery with calcium and/or vitamin D deficiency.
KEYWORDS: bariatric surgery, calcium absorption, vitamin D status, bone health
Bariatric Times. 2020;17(6):10–14
It is widely known that systems within the body comprises dynamic and active cells, and bone tissue is no exception. Cellular development and reconstruction within the bodily systems depend on several factors, including nutritional status. The bone matrix not only stores certain micronutrients, but also depends on them for normal structure and function, and exchanges minerals, such as calcium, with the blood and extracellular fluids as needed.1 All bodily systems are susceptible to injury, disorders, and disease.
Bone tissue is dynamic—it continually renews itself by removing old bone through osteoclasts (bone resorption) and building new bone through osteoblasts. The balance between osteoclast and osteoblast activity is crucial to maintain bone mineral density. When this balance is skewed and bone turnover exceeds the rate of bone growth, a loss of density and strength can occur, leading to osteopenia, osteomalacia, osteoporosis, and risk of bone fracture.2
Bariatric surgery is associated with a decrease in bone mass and an increase in fracture risk.3 Bone loss after bariatric surgery has been an area of interest in research for over two decades, with an influx of publications on the subject over the last 10 years.4 The majority of studies show that bone loss and fracture risk following bariatric surgery present at a faster rate than both controls and compared to intensive medical treatment.4 Bariatric surgery changes the gastrointestinal (GI) tract in ways that cause an immediate and unfavorable effect on its ability to absorb many nutrients needed for healthy bone remodeling, and we are beginning to understand that bariatric surgery causes significant and ongoing bone loss up to at least 10 years postoperatively, which is the current length of time that it has been studied.5,6
Some proposed mechanisms of this are hormonal changes, including, abnormalities in calciotropic hormones and changes in gut hormones, mechanical unloading of body weight, and the loss of lean mass along with a loss of fat mass with an increase in bone marrow fat.5,7 The focus of this article will the role that calcium and vitamin D play on bone health following bariatric surgery, while highlighting that nutrient deficiencies are just one part of the bone loss puzzle. For an up-to-date, extensive review of bone health following bariatric surgery, an article by Saad et al4 was published this year. See the “Clinical Considerations” Box on the next page for additional recommended reading.8,9
Bone Loss After Bariatric Surgery
The growing body of literature continues to show that the two most common bariatric surgery procedures, the Roux-en-Y gastric bypass (RYGB) and the vertical sleeve gastrectomy (VSG)can result in persistent and ongoing bone loss.10 Data on purely restrictive procedures, including the adjustable gastric band (AGB), have shown mixed results on the impact of bone health, while procedures with the greatest degree of malabsorption, such as the biliopancreatic diversion with or without duodenal switch (BPD-DS), have the least amount of published data. The results indicate that these patients are at the greatest risk of bone loss.4
Excessive bone resorption has been found to start as early as 10 days postoperatively in patients following RYGB surgery, by evidence of an increase in serum C-terminal telopeptide Type 1 collagen (CTX), which has been found to remain elevated for 1 to 2 years and slowly decline over the second postoperative year, despite weight stabilization.4
Bone loss after bariatric surgery is a concern because of the risk of increased skeletal fragility in a patient population primarly around 40 years of age, a time when bone tissue production has already begun to decline, compounding the risk for fracture.2 An interesting area of study is the bone health of adolescents who have had bariatric surgery—limited data exist at this time. While increased body weight has generally been viewed as bone protective, it is unclear if excess adiposity is beneficial or unfavorable to bone health. More recent studies have found that the relationship between body mass and skeletal status is complex and that excess weight due to excess adipose tissue might be detrimental to bone, potentially due to a disruption of bone regulating hormones.11,12
Abnormalities in vitamin D metabolism, calcium absorption, and parathyroid hormone (PTH) concentrations are common after bariatric surgery.6 This combination, along with the dramatic weight loss that typically occurs after surgery, increases the risk of bone abnormalities, fractures, and metabolic bone disease.6 Even prior to surgery, research has indicated that people with obesity have lower serum vitamin D levels, lower calcium intake, and higher PTH concentrations than age- and sex-matched individuals.13 In studies evaluating fracture risk for postsurgical patients compared to controls with obesity, fracture risk for BPD-DS was shown to increase by 60 percent and for RYGB by 47 percent.4
Nutrients with a Role in the Bone Matrix
Calcium. Calcium is the most abundant mineral in the body and is often associated with the formation and metabolism of bone. Calcium hydroxyapatite in the bones and teeth makes up around 99 percent of total body calcium, where it provides hard tissue its strength. The remaining one percent of calcium is found in the blood and extracellular fluids, where it plays a vital role in proper functioning of the circulatory system, muscles, and other tissues. Serum calcium is critical for mediating vascular contraction and vasodilatation, nerve transmission, intracellular signaling, and hormonal secretion. Bone tissue store calcium for these crucial metabolic needs through the process of bone remodeling.1
Calcium intake and needs. Adequate calcium intake across the lifespan is critical, particularly in the prepubertal and adolescent stages. Calcium is required for optimal bone mass and density gains, especially in female patients.1 Under healthy circumstances, typical bone remodeling patterns change during different cycles of the lifespan: bone formation is greater than resorption in periods of growth, such as childhood and adolescence years, generally equal during adult years, and, during the older years of life, bone resorption is greater than growth.14
Data suggest that calcium intake during all stages of life is less than desirable for the majority of people in the United States (US), potentially increasing the risk for lower-than-expected bone mass and density. The National Health and Nutrition Examination Survey (NHANES) 2003 to 2006 data found that certain groups of people fell 50 percent below their respective estimated average requirement (EAR) of calcium. These included girls aged 9 to 18 years, women aged 51 to 70 years, and both men and women older than 70 years.15
US population data from the NHANES 2001 to 2008 found that intakes of many micronutrients are even lower among people with obesity. More than 40 percent of US adults take in less than the recommended dietary allowances (RDAs) of calcium, magnesium, and vitamin D; in adults with obesity, intakes of micronutrients were found to be 5 to 12 percent lower than adults with normal weight.16 See Table 1 for the RDAs and tolerable upper intake levels (ULs) for adults for calcium.17
Calcium requirements increase with age, especially among postmenopausal women, due to a decrease in estrogen levels. Estrogen plays a role in bone resorption and contributes to the efficiency of intestinal absorption and renal conservation of calcium in female patients.18 In a large cohort of postmenopausal women, those with lower fractional calcium absorption were more likely to experience a hip fracture, and this association was higher for those with low calcium intakes.19
Calcium absorption, transport, and excretion. Not only are patients who undergo bariatric surgery at risk for low calcium intake prior to surgery, but calcium intake and the bodyu’s ability to absorb it have been shown to decrease after surgery.12
In an unaltered GI, calcium can be absorbed throughout the length of the small intestine and in small amounts in the colon.1 Calcium is most rapidly absorbed (about 80% of intake) in the duodenum and proximal jejunum via active transport and is dependent upon an acidic environment and vitamin D status.1,20 Passive transfer occurs along the length of the small intestine, independent of vitamin D status, and is primarily dependent on the amount of ionized calcium found in the gut, whether from dietary or supplemental sources.1
Estimates of calcium absorption in healthy adults is around 30 percent of intake.1 Factors that influence calcium bioavailability include the body’s calcium and vitamin D status, presence of other dietary enhancers (lactose) or inhibitors (oxalates found in many vegetables and fruits or phytates found in whole grains), an acidic environment, and certain medications.1
Calcium status assessment. Total serum calcium is very tightly regulated and does not fluctuate with changes in dietary intakes. Any change in serum levels signals a cascade of calcium regulating hormones to release stored calcium from the bone to maintain serum levels; thus, low dietary intake, poor absorption of calcium or a combination of both will not cause the serum level of calcium to fall out of normal range.1 Total serum calcium consists of three entities: free or ionized, (47.6%), albumin-bound (46%), and complexed to an anion (6.4%).1 For these reasons, assessing calcium status can be difficult. One of the most sensitive indicators of calcium intake is urinary calcium excretion; however, this is not a typical order because it requires a 24-hour urine collection.17
Impact of bariatric surgery on calcium absorption. RYGB and VSG impact nutrient absorption in several ways. Both procedures reduce the amount of food that can be consumed at one time, as well as alter the GI tract, especially through reduction of hydrochloric acid (HCl), which is essential in the digestion process via its role in the dissolution of many micronutrients necessary for bone formation (calcium, magnesium, and vitamins ADK) and bone remodeling (vitamin E, B-vitamins, essential fatty acids, vitamin C, boron, and several other trace minerals).8,21 In particular, HCl is necessary for solubilization of calcium, which allows its (active) absorption.1 Important to note: in RYGB procedures, the duodenum and proximal jejunum are bypassed, which are the only active sites of calcium absorption in the GI tract.
Calcium absorption after RYGB surgery. Fractional calcium absorption (FCA), a measure of the amount of calcium absorbed within the intestine, was evaluated in a prospective cohort study of 33 patients who underwent RYGB (58% premenopausal women; 18% postmenopausal women; 24% men), prior to surgery and then again six months postoperatively.22 The study group was carefully monitored throughout the study, and vitamin D and chewable calcium citrate were provided to the patients for the duration of the study. Investigators tailored daily vitamin D recommendations based on each patient’s serum 25(OH)D levels to maintain levels of at least 30ng/mL (mean cholecalciferol [Vitamin D3] supplement dose at the 6-month postoperative visit was 3121±1111 IU). The investigators also individualized daily calcium supplement recommendations alongside dietary intake measures to provide a total (dietary and supplemental) of 1,200mg calcium daily throughout the study (the published article did not provide average supplemental calcium dosing).22
Prior to the preoperative investigation time point, 25(OH)D levels were repleted from 23.6ng/mL at initial enrollment to 41.0ng/mL, to ensure adequate vitamin D status. After an overnight fast, study participants were given a test meal that contained 300mg of calcium, which was consumed in its entirety in a laboratory setting. Results prior to surgery found that FCA was 32.7 percent, which is within the expected range. Six months after surgery, the participants consumed the identical test meal in its entirety containing 300mg of calcium in a laboratory setting and consumed the entire meal. The decline in FCA was dramatic at 6.9 percent, despite maintaining 25 OHD status at greater than 30ng/mL and a recommended intake of 1,200mg of calcium each day. Additional noteworthy findings included a decrease in 24-hour urinary calcium, areal bone mineral density (BMD) at the proximal femur, volumetric BMD at the spine, and an increase in PTH and bone turnover markers. The study was limited by its short duration of six months and observational design.22
Calcium absorption after RYGB or VSG. A two-year prospective study on calcium absorption in premenopausal patients who underwent RYGB or VSG sought to determine if patients who underwent VSG experienced a decline in FCA at the same rate as patients who underwent RYGB.23 The authors hypothesized that because the small intestine is not rerouted during a VSG procedure, a decline in FCA markers would be less in patients who had undergone this procedure, compared to patients who had undergone RYGB. Additional aims of this study were to determine modifications of BMD, intakes of calcium and vitamin D, vitamin D status, and PTH concentrations up to 24 months after surgery. The participants were required to maintain a record of vitamin and mineral supplementations throughout the study period, in addition to three-day food records, and then participate in an interview by a dietitian for validation of the self-reported nutrient intakes.23
The study began with 58 premenopausal women (n=26 VSG; n=32 RYGB) and concluded, at two years, with 34 patients (n=14 VSG; n=21 RYGB) who were available for analysis.23 FCA was measured prior to surgery and again at Months 12 and 24 postoperation, using a standard liquid test meal at each time point, comprising 100 calories, 7.6g protein, 8.4g carbohydrates, 4.0g fat, 40mg phytate, and 17.6mg calcium. The authors aimed to specifically evaluate active calcium absorption by providing a low-dose calcium load in the test meal (where one might assume that study investigators wanted to evaluate total calcium absorption through active and passive means by providing a larger test dose of calcium).23
Calcium absorption was significantly reduced for both surgical procedures at each time point after surgery, and the magnitude of the reduction was not statistically significant between surgery groups.23 Calcium absorption rates in the RYGB group prior to surgery, postoperative Month 12, and postoperative Month 24 were noted to be approximately 37 percent, 21 percent, and 18 percent, respectively, while the calcium absorption rates noted in the VSG group were approximately 41 percent, 27 percent, and 17 percent, respectively.
A limitation of this study was the small sample size. However, the authors concluded that both of the surgical procedures increased the risk of unfavorable calcium absorption and compromised calcium homeostasis in premenopausal women who undergo RYGB or VGB surgery, stating that the study reinforced the importance of high-calcium and vitamin D supplements following bariatric surgery.
Identification of calcium deficiency. Serum calcium tests are unreliable in detecting calcium deficiency due to the body pulling stored calcium from the bone to maintain normal limits to prevent a drop in serum calcium. Thus, unlike deficiencies of other micronutrients, calcium deficiency needs to be assessed by a variety of clinical methods. Some of these methods, including bone density studies, are only recommended every few years after surgery, and data reporting on the percentage of patients who have these studies completed are lacking. These factors present diagnostic challenges in the identification of potential calcium deficiency. Table 2 provides various screening measures for determining calcium status.
Vitamin D. Vitamin D is a fat-soluble vitamin that is only found naturally in a few foods. Vitamin D is primarily obtained by way of the diet through consumption of fortified foods and/or dietary supplements. Vitamin D is primarily produced endogenously via ultraviolet (UV) rays that hit the skin and activate vitamin D synthesis, which has led some scientists to classify it as a prohormone because it is produced by the body. Vitamin D is biologically inert and must undergo a two-step activation process via the liver and kidneys to become physiologically active.24
Vitamin D plays many roles within the body, including an influence on cellular growth, proliferation, differentiation, and apoptosis; roles in neuromuscular and immune function; and reduction of inflammation. Perhaps its most significant role in the body is bone strength development through its facilitation of calcium absorption and assistance of PTH in maintaining adequate serum calcium and phosphate concentrations, which enable normal mineralization of bone. Vitamin D is also necessary for bone growth and remodeling, and without sufficient vitamin D availability, bones can become thin, brittle, or misshapen.25
Vitamin D intake and needs. The body can produce some of its own vitamin D through sun exposure. However, season, time of day, cloud cover, smog, skin melanin content, and sunscreen are factors that affect UV radiation exposure, and subsequently impact host vitamin D synthesis.25 The greatest naturally occurring sources of vitamin D from food, primarily in the form of vitamin D3, include the flesh of fatty fish, beef liver, cheese, and egg yolks. Mushrooms are sources of vitamin D2.26 Fortified foods, such as milk, breakfast cereal, and yogurt, provide most of the vitamin D in the American diet.27
The RDAs for vitamin D do not vary as widely according to age and sex of the individual. Everyone from the age of 1 to 70 years is recommended to consume 15mcg (600 IU) of vitamin D per day, and for those above 70 years of age, the recommendation is 20mcg (800 IU).28 The NHANES 2005 to 2006 data found that the average intake levels from food alone for male individuals was approximately 59 percent below the RDA, and for female individuals, approximately 65 percent below the RDA. When dietary supplements were taken into consideration, these intake levels rose. It is estimated that 37 percent of the US population uses a supplement containing vitamin D.15
Vitamin D deficiency causes abnormalities in calcium, phosphorus, and bone metabolism. Severe and prolonged deficiency can lead to metabolic bone disease, and in recent years has been associated with fractures, falls, certain types of cancer, diabetes, cardiovascular disease, and depression, though its role in these health conditions lack general consensus due to the cutoff-point variance seen in many studies when attempting to define optimal vitamin D levels.29
Vitamin D absorption, transport, and excretion. Ingested vitamin D is absorbed primarily in the jejunum and ileum, along with lipids, by micelle-dependent diffusion and is then picked up by chylomicrons and transferred in the plasma via vitamin D-binding protein (DBP).1 Interestingly, DBP is dependent on vitamin D for formation, which means that in a vitamin D-deficient state, the body’s ability to create the protein that is needed to transport vitamin D to tissues for metabolism or utilization will be limited.30
Given that absorption of vitamin D in the small intestine depends on bile salt interaction, any condition that limits the interaction of ingested food with the common bile duct can result in fat malabsorption and lower the ability of the body to absorb fat-soluble vitamins.30 The body recycles vitamin D from bile, which is the primary route of excretion, placing patients who have had BPD-DS at an even further disadvantage due to a shortened common channel, which provides little opportunity for this recycling to occur.
How to assess vitamin D status. Serum concentrations of 25(OH)D are the preferred method of assessing vitamin D status; however, optimal serum levels for bone and general health have not been established and are likely to vary at each stage of life and health status.25,31 Serum 25(OH)D has a 15-day half-life and reflects cutaneous factors and the amount of vitamin D obtained from the diet.32 Serum 25(OH)D serves as a biomarker of exposure but does not indicate the amount of vitamin D stored in body tissue.28
Research has indicated that serum 25(OH)D levels increase in response to increased vitamin D intake, but the increase is nonlinear and tends to depend on baseline serum levels and duration of the supplementation.28 Published guidelines for the general population, as well as the bariatric surgery population, suggest that the serum concentration of 25(OH)D should be maintained at greater than 30ng/mL (>75 nmol/L) to maximize the effect of calcium, bone, and muscle metabolism.33,34 Additionally, serum concentrations of greater than 50ng/mL (>125nmol/L) are associated with potential adverse effects.24
A body mass index (BMI) of at least 30kg/m2 is associated with lower serum 25(OH)D levels when compared to people without obesity, and this is likely due to many factors. Potential contributors include the sequestering of vitamin D in subcutaneous fat, which alters its release into circulation (See Clinical Considerations Box on the previous page), potential diseases of the liver or kidneys (e.g., nonalcoholic fatty liver disease or chronic kidney disease from complications of diabetes or hypertension), potential genetic variations in the function of DBP or in vitamin D receptors that bind and hold vitamin D, and volumetric dilution.35–38
Impact of bariatric surgery on vitamin D.
Studies assessing vitamin D absorption following bariatric surgery are sparse; however, vitamin D deficiency occurs at high rates both prior to and after surgery. Measuring vitamin D absorption is not as straightforward as absorption tests for calcium for several reasons, including the endogenous production of vitamin D, its transport in the lymphatic system, and the length of time it takes the body to convert administered cholecalciferol into 25(OH)D.39 It is estimated that it takes about 14 days from the time of ingested supplemental vitamin D3 to be converted to circulating 25(OH)D, and in people with obesity this process is speculated to take longer.40
It stands to reason that intestinal absorption of vitamin D is reduced after bariatric procedures that bypass the upper portion of the small intestine due to lack of interaction with bile salts and subsequent micelle formation, on which vitamin D is dependent for absorption, due to a delay in the blend of nutrients. Other contributing factors to vitamin D deficiency after bariatric surgery include potential existing preoperative deficiency, inadequate vitamin D supplementation, and intestinal bacterial overgrowth, which might interfere with vitamin D absorption.41
Identification of vitamin D deficiency. Vitamin D deficiency is one of the most common nutrient deficiencies both before and after bariatric surgery33 and is often asymptomatic. Serum marker assessment of vitamin D status can be easily evaluated by the HCP, and the following categories are provided to help guide supplemental recommendations:33,42
Deficient: less than 20ng/mL (50nmol/L)
Insufficient: 20 to 30ng/mL (50–75nmol/L)
Adequate: greater than 30ng/mL (>75nmol/L)
Potentially harmful: greater than 60ng/mL (>150nmol/L)
Over supplementation of vitamin D can cause toxicity; however, this is extremely rare and generally only occurs after ingestion of large doses of vitamin D (>10,000 IU per day) for prolonged periods of time in patients with unaltered anatomy.43 Risks of extended 25(OH)D levels in the general population include calcification of soft tissue. However, to date, there have not been reports of this in bariatric surgery literature.
Vitamin D supplement recommendations. Optimal dosing strategies for repletion and maintenance of adequate 25(OH)D levels have not been identified at this point in time.44 Bariatric clinical guidelines suggest supplementing patients on an individual bases to maintain serum levels of 25(OH)D above 30ng/mL,33,45 regardless of procedure type.
The typical recommended dose to achieve this is 2,000 to 3,000 IU (50–75mcg) per day;45 however, there is great individual variability in response to this dose. Ongoing research is underway in the general population to develop an algorithm to help guide clinicians on dosing strategies using body weight and current serum levels, but until then, clinicians must work closely with patients and tailor recommendations based on response to treatment, which is often highly individualized.
Clinical practice guidelines vary regarding suggestions for repletion of vitamin D, but proposed dosing strategies include:
At least 3,000 IU and as high as 6,000 IU per day,33,45 OR 50,000 IU 1 to 3 times per week33,45
50,000 IU per week for 8 to 12 weeks46,47
6,000 to 10,000 IU per day (until >30ng/mL); then maintenance dose of 3,000 to 6,000 IU per day34
Severe: 50,000-150,000 IU per day with calcitriol 0.25 to 0.5mcg48
The HCP needs to be mindful that large doses of vitamin D are meant to replete a deficiency and need to closely monitor the patient and evaluate serum trends to ensure vitamin D status is improving and to avoid oversupplementation. The source of vitamin D for repletion can be vitamin D2 (ergocalciferol) or D3 (cholecalciferol), as both have been found to be efficacious
depending on the dose use. However, vitamin D3 is recommended because it is a more potent source.33
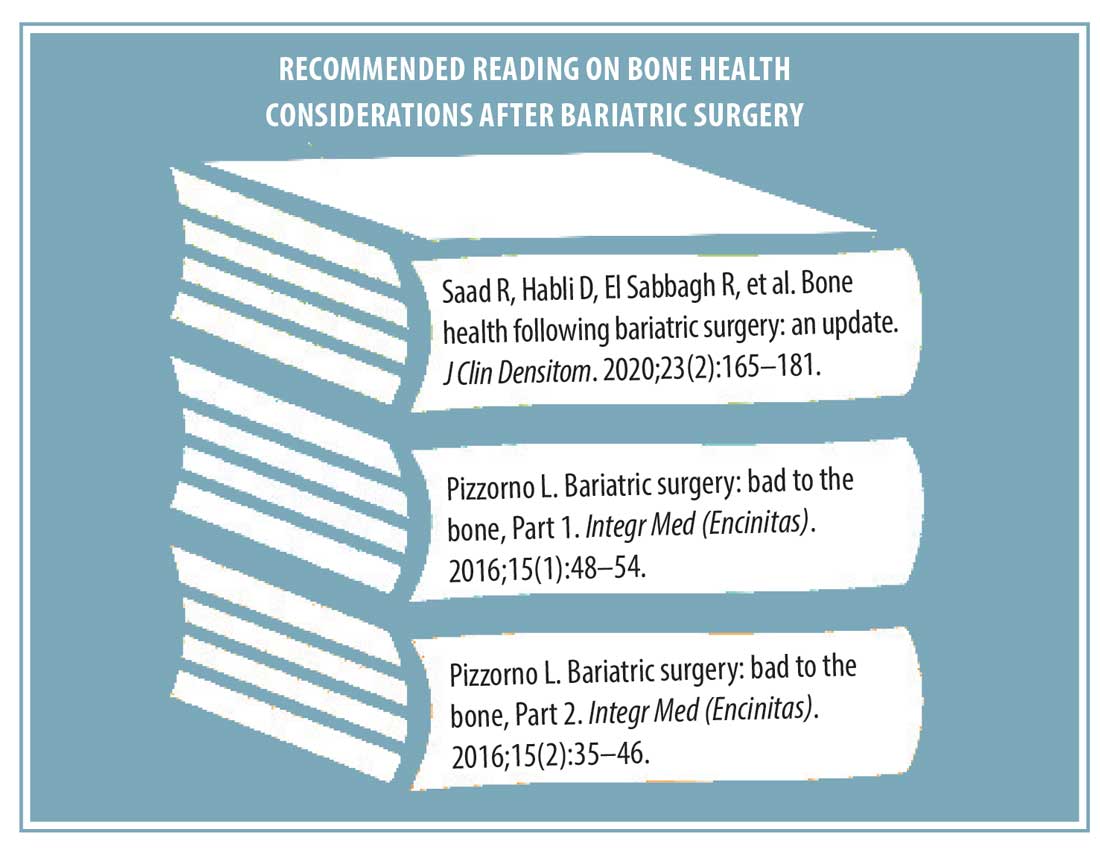
Conclusion
There are many micronutrients, in addition to calcium and vitamin D, and several other factors that play important roles in bone health and skeletal remodeling after bariatric surgery, including vitamin K, magnesium, protein intake, physical activity levels, and hormonal changes; however, review of these are beyond the scope of this article. However, due to the high incidence of calcium and vitamin D abnormalities after bariatric surgery, it is imperative that bariatric clinicians spend time educating patients on the importance of lifelong follow-up care, including complete nutritional evaluations (with serum marker evaluation) and bone density screening to protect their skeletal system.
References
- Mahan L, Escott-Stump S. Krause’s Food, Nutrition, and Diet Therapy 10th edition. Philadelphia, PA: W.B. Saunders Company, 2000.
- Liu C, Wu D, Zhang J, et al. Changes in bone metabolism in morbidly obese patients after bariatric surgery: a meta-analysis. Obes Surg. 2016;26:61–70.
- Folli F, Sabowitz B, Schwesinger W, et al. Bariatric surgery and bone disease: from clinical perspective to molecular insights. Int J Obes. 2012;36(11):1373–1379.
- Saad R, Habli D, El Sabbagh R, et al. Bone health following bariatric surgery: an update. J Clin Densitom. 2020;23(2):165–181.
- Stein E, Silverberg S. Bone loss after bariatric surgery: causes, consequences, and management. Lancet Diabetes Endocrinol. 2014;2(2):165–174.
- Pizzorno L. Bariatric surgery: bad to the bone, Part 1. Integr Med (Encinitas). 2016;15(1):48–54.
- Gagnon C, Schafer AL. Bone health after bariatric surgery. JBMR Plus. 2018;2(3):121–133.
- Piccolo B, Adams S, Van Loan M, et al. Association between subcutaneous white adipose tissue and serum 25-hydroxyvitamin D in overweight and obese adults. Nutrients. 2013;5(9):3352–3366.
- Lin E, Armstrong-Moore D, Liang Z, et al. Contribution of adipose tissue to plasma 25-hydroxyvitamin D concentrations during weight loss following gastric bypass surgery. Obesity. 2011;19(3):588–594.
- Pizzorno L. Bariatric surgery: bad to the bone, Part 2. Integr Med (Encinitas). 2016;15(2):35–46.
- Cao J. Effects of obesity on bone metabolism. J Orthop Surg Res. 2011;6:30.
- Ben-Porat T, Elazary R, Sherf-Dagan S, et al. Bone health following bariatric surgery: implications for management strategies to attenuate bone loss. Adv Nutr. 2018;9:114–127.
- Snijder M, van Dam R, Visser M, et al. Adiposity in relation to vitamin D status and parathyroid hormone levels: a population-based study in older men and women. J Clin Endo Metab. 2005;90(7):4119–4123.
- Ross A, Abrams S, Aloia J, et al. Committee to Review Dietary Reference Intakes for Vitamin D and Calcium, Food and Nutrition Board, Institute of Medicine. Dietary Reference Intakes for Calcium and Vitamin D. Washington, DC: National Academy Press, 2010.
- Bailey R, Dodd K, Goldman J, et al. Estimation of total usual calcium and vitamin D intakes in the United States. J Nutr. 2010;140(4)817–822.
- Agarwal S, Reider C, Brooks JR, et al. Comparison of prevalence of inadequate nutrient intake based on body weight status of adults in the United States; an analysis of NHANES 2001-2008. J Am Coll Nutr. 2015;34(2):126–134.
- NIH Office of Dietary Supplements. Office of Dietary Supplements – Calcium. https://ods.od.nih.gov/factsheets/Calcium-HealthProfessional/#en1. Accessed February 7, 2020.
- Gallagher J, Lawrence Riggs B, Deluca H. Effect of estrogen on calcium absorption and serum vitamin D metabolites in postmenopausal osteoporosis. J Clin Endocrinol Metab.1980;51(6):1359–1364.
- Ensrud K, Duong T, Cauley J, et al. Low fractional calcium absorption increases the risk for hip fracture in women with low calcium intake. Study of osteoporotic fractures research group. Ann Intern Med. 2000;132:345–353.
- Carlin A, Rao D, Yager K, et al. Effect of gastric bypass surgery on vitamin D nutritional status. Surg Obes Relat Dis. 2006;2(6):638–642.
- Via M, Mechanick J. Nutritional and micronutrient care of bariatric surgery patients: current evidence update. Curr Obes Rep. 2017;6:286–296.
- Schafer A, Weaver C, Black D, et al. Intestinal calcium absorption decreases dramatically after gastric bypass surgery despite optimization of vitamin D status. J Bone Miner Res. 2015;30(8):1377–1385.
- Carrasco F, Basfifer K, Rojas P, et al. Calcium absorption may be affected after either sleeve gastrectomy or Roux-en-Y gastric bypass in premenopausal women: a 2-year prospective study. Am J Clin Nutr. 2018;108:24–32.
- Institute of Medicine, Food and Nutrition Board. Dietary reference intakes for calcium and vitamin D. Washington, DC: National Academy Press, 2010.
- Cranney A, Horsley T, O’Donnell S, et al. Effectiveness and safety of vitamin D in relation to bone health. Evid Rep Technol Assess (Full Rep). 2007;158:1–235.
- Mattila P, Piironen V, Uusi-Rauva E, et al. Vitamin D contents in edible mushrooms. J Agric Food Chem. 1994;42:2449–2453.
- Calvo M, Whiting S, Barton C. Vitamin D fortification in the United States and Canada: current status and data needs. Am J Clin Nutr. 2004;80:1710S–1716S.
- NIH Office of Dietary Supplements. Office of Dietary Supplements – Vitamin D. https://ods.od.nih.gov/factsheets/VitaminD-HealthProfessional/. Accessed: February 7, 2020.
- LeFevre M, Chou R, Zakher B, et al. Screening for vitamin D deficiency in adults: US preventative services task force recommendation statement. Ann Intern Med. 2015;162:133–141.
- Jacques J. Micronutrition for the Weight Loss Surgery Patient. Edgemont, PA: Matrix Medical Communications, 2006.
- Holick M. Vitamin D deficiency. N Engl J Med. 2007;357:266–281.
- Jones G. Pharmacokinetics of vitamin D toxicity. Am J Clin Nutr. 2008;88:582S–586S.
- Parrott J, Frank L, Rabena R, et al. American Society for Metabolic and Bariatric Surgery Integrated Health Nutritional Guidelines for the Surgical Weight Loss Patient 2016 Update: Micronutrients. Surg Obes Relat Dis. 2016;12:955–959.
- Holick M, Binkley N, Bischoff-Ferrari H, et al. Evaluation, treatment, and prevention of vitamin D deficiency: an Endocrine Society Clinical Practice Guideline. J Clin Endocrinol Metab. 2011;96:1911–1930.
- Gozdzik A, Zhu J, Wong BYL, et al. Association of vitamin D binding protein (VDBP) polymorphisms and serum 25(OH)D concentrations in a sample of young Canadian adults of different ancestry. J Steroid Biocehm Mol Biol. 2011;127(3-5):405–412.
- Drincic A, Armas L, Van Diest E, et al. Volumetric dilution, rather than sequestration best explains the low vitamin D status of obesity. Obesity. 2012;20(7):1444–1448.
- Gonzalez E, Sachdeva A, Oliver D, et al. Vitamin D insufficiency and deficiency in chronic kidney disease. Am J Nephrol. 2004;24(5):503–510.
- Eliades M, Hernaez R, Spyrou E, et al. Meta-analysis: vitamin D and non-alcoholic fatty liver disease. Aliment Pharmacol Ther. 2013:38(3):246–254.
- Aarts E, van Groningen L, Horst R, et al. Vitamin D absorption: consequences of bypass surgery. Eur J Endocinol. 2011;164:827–832.
- Heaney R, Armas L, Shary J, et al. 25-hydroxylation of vitamin D3: relation to circulating vitamin D3 under various input conditions. Am J Clin Nutr. 2008;87:1738–1742.
- Lespessailles E, Toumi H. Vitamin D alteration associated with obesity and bariatric surgery. Exp Biol Med (Maywood). 2017;242:1086–1094.
- Holick M. Vitamin D status: measurement, interpretation and clinical application. Ann Epidemiol. 2009;19(2):73–78.
- Kennel KA, Drake MT, Hurley DL. Vitamin D deficiency in adults: when to test and how to treat. Mayo Clin Proc. 2010;85:752–758.
- Chakhtoura M, Nakhoul N, Akl EA, et al. Guidelines on vitamin D replacement in bariatric surgery: identification and systematic appraisal. Metabolism. 2016;65(4):586–597.
- Mechanick J, Apovian C, Brethaur S, et al. Clinical practice guidelines for the perioperative nutrition, metabolic, and nonsurgical support of patients undergoing bariatric procedures – 2019 update: cosponsored by American Association of Clinical Endocrinologists/American College of Endocrinology, The Obesity Society, American Society for Metabolic & Bariatric Surgery, Obesity Medicine Association, and American Society of Anesthesiologists. Surg Obes Relat Dis. 2020;16(2):175–247.
- Cummings S, Isom, K. WM DPG AND Pocket Guide to Bariatric Surgery Edition 2: Appendix D & E.
- Bays H, Jones P, Jacobson T, et al. Lipids and bariatric procedures Parts 1 & 2: Scientific statement from the National Lipid Association, ASMbariatric surgery, OMA: Executive Summary. J Clin Lipid. 2016;10:15–32.
- Stein J, Stier C, Raab H, et al. Review article: the nutritional and pharmacological Consequences of obesity surgery. Al Pharm Ther. 2014;40:582–609.
Category: Past Articles, Review